Physical fitness
Physical fitness is an umbrella that encapsulates our ability to perform tasks that include sports but also our regular day-to-day. It is determined by a spectrum of activity that spans from physical exercise, to recovery to nutrition. In terms of physical exercise, working out promotes better musculoskeletal and cardiovascular resistance, lessening the odds of disease or injury (1). Moderate regular exercise also benefits tissue as well as nervous (2) and digestive systems (3).
Aerobic Exercise
According to the American College of Sports Medicine (ACSM), aerobic exercise is defined as any activity that uses large muscle groups, can be maintained continuously and is rhythmic in nature( 4). It is mentioned that during activities such as cycling, dancing, hiking, jogging, swimming and walking, the aerobic capacity allows the cardiovascular and musculoskeletal systems to utilize oxygen (5)(6). This type of exercise triggers muscle groups into extracting Adenosine triphosphate (or ATP) as an energy source.
Anaerobic Exercise
Alternately, anaerobic exercise involves shorter duration but with bursts of intense physical activity, such as high-intensity interval training (HIIT) or body building. This type of exercise relies on glycogen from contracting muscles as an energy source ( 5), independent of oxygen inhalation. Because of the lack of oxygen, ATP is produced instead through glycolysis; a process of breaking down glucose to create ATP. Glycolysis can happen with oxygen (aerobic glycolysis) or without oxygen (anaerobic glycolysis). Fermentation produces ATP by the degradation of organic nutrients (carbohydrates, proteins and fat) anaerobically. Muscles mostly use glucose (sugar) as fuel for contraction (or in other words, for creating ATP, a necessary ingredient for muscle contraction). This results in significantly less ATP production as well as increased lactic acid levels and metabolic acidosis ultimately resulting in muscle soreness and stiffness, depending on the fitness level.
Exercise and Hormetic Stressors
The effects of exercise on the human body are hormetic. Hormesis is the adaptive response of cells and organisms to moderate and/or intermittent stress exposure resulting in increased resiliency of bodily systems (2).
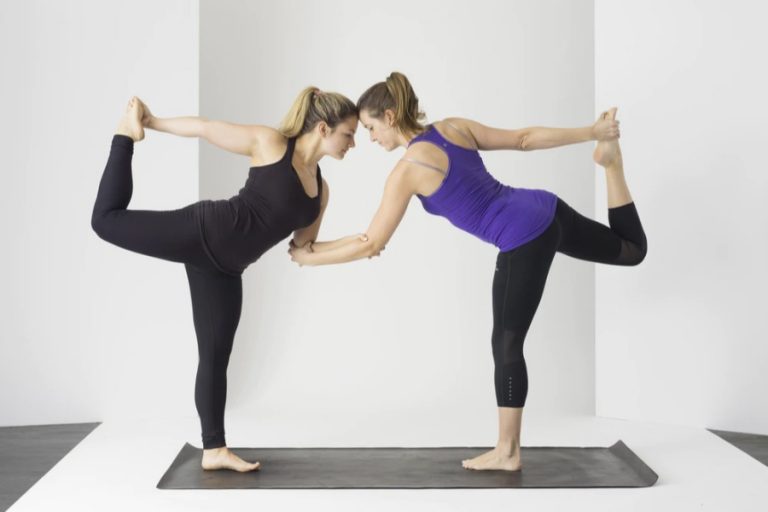
FATIGUE-RECOVERY FACTORS
Muscle Damage
High-intensity anaerobic and resistance exercise can greatly agitate skeletal muscle and connective tissue (8). Until these components are recovered in full, sensations of soreness and pain will persist, as muscle performance abilities are reduced. The damage in the muscles in turn disrupts the transport of blood glucose, which lowers the capacity to replenish glycogen stores (9), affecting muscle activation and overall performance.
Decreased Substrates
Powerlifting, sprinting or other intense muscle contracting exercises are fuelled by phosphocreatine (PCr) stores in the muscles. Unlike the tendency of aerobic contractions to utilize oxygen as an energy source, phosphocreatine produces energy without the help of oxygen. To begin with, the stores of PCr in skeletal muscle is limited, and within 10 seconds of initiating high intensity workouts, can be rapidly exhausted (10). Recovery from this loss is vital, as prolonged exposure to this type of exercise can also lead to the depletion of primary substrates for glycogen. Unless glycogen provisions are sufficiently restored before the next exercise session, performance will be affected (8).
High intensity exercise can lead to the accumulation of lactate and protons in the cells, both of which impair ATP recovery and skeletal muscle contraction (4). Lactate specifically encumbers muscle contraction by interfering with the electrical stimulus. Muscle pH is also resultingly decreased by the accumulation of protons (both of them). The accumulation of both, also known as Cellular Acidosis, impairs creatine phosphate (CrP) replenishment. In other words, recovery from cellular acidosis is vital to restoring the capacities of the phosphagen system and glycolysis to produce ATP (5).
General Adaptation Syndrome (GAS)
Bodily responses to stressors, in this case exercise, are explained through Selye’s General Adaptation Syndrome (GAS). GAS dictates that biological systems will adapt to any stressors they might experience to meet the demands of these stressors (16)(17)(18). According to Selye, this is accomplished in three phases; the initial reaction to the stressor is termed the alarm/reaction phase where the athlete may experience stiffness, soreness, or a small drop in performance from fatigue after the training session. Heart rate and blood pressure surges, as well as cortisol and adrenaline level increases are expected. The second phase was termed the resistance phase, in which the body responds to stressors by adapting to new stress with less soreness, stiffness, more tolerance to activity, and improved performance. This is considered to occur at a level greater than that demanded by the stressor, termed supercompensation. The final phase occurs if the stressor goes on longer than the organism can adapt, and exhaustion results, whereby the athlete may experience lowered performance in training or symptoms of overtraining (16). Therefore, readiness for exercise is the result of the relationship between fitness level and fatigue (18)(20). To achieve optimal results in the adaptation of the neuromuscular system to training-induced stress, alterations in volume and intensity are necessary. Demanding exercises routines allow the neuromuscular system to adapt by amplifying muscle performance, while also simultaneously increasing physical, mental and metabolic costs of recovery. Without overload factors, the system needn’t adapt to stressors and increases in the desired outcome will eventually halt (15)(19)(20). However, if the overload is too high, the degree of physiological damage will affect readiness for training. What is suggested is the implementation of a systematically planned program to avoid such results, as the expected load for neuromuscular systems to adapt and minimize fatigue for varies.
Post-Exercise Recovery
Within the exercise training paradigm, recovery remains a vital component, especially in terms of optimal performance and continued improvement. With the appropriate rate of recovery, higher training intensity is possible without the detrimental effects of overtraining (1).
Supercompensation Theory
To maintain a state of homeostasis, the body attempts to adapt to environmental stressors. Training is therefore the manipulation of stress application, and the body’s adaptation to said stress is an effort to maintain homeostasis. The desired adaptive response for training is also termed supercompensation, refering to a post-exercise state during which the performance of a desired fitness component, such as endurance, speed or strength, is elevated compared to the baseline. Following training sessions, an athlete may show decreased performance level – measurable by fitness components. Diminished performance is caused by fatigue, directly due to the training stimulus. After a recovery period, the body then adapts to said stimulus in order to prepare itself for future training by increasing its capacity to tolerate the stimulus, perhaps by becoming faster, stronger and so on. Therefore, adequate post-exercise recovery is essential in long-term performance enhancement. If an athlete does not properly recover before their next training session, it may result in decreased performance. Long-term recovery deficiencies may also result in over-training syndrome (11)(13).
Metabolic Responses and Adaptations to Exercise
The principle of training specificity states that the physiological and metabolic responses and adaptations to exercise are specific to the type of exercise and muscle groups involved.
Increased VO2max
Increased maximal oxygen uptake (VO2max) is linked with heightened performance levels. VO2max plays an integral role in post-exercise recovery in endurance-related activity; research shows that individuals with greater VO2max recover quicker between sprints (7) and have superior performance in later bursts of sprints. Individuals with higher VO2max levels also replenish CrP stores faster (5).
Increased Buffering Capacity
As mentioned before, an accumulation of proton molecules in the cells can disrupt muscle pH and impair skeletal muscle function. Proton accumulation, or acidosis is combated by buffering-capacity system in the cellular environment. This system in untrained individuals is easily overwhelmed during high-intensity activity. Adequate training cultivates increased buffering-capacity, which contributes to more efficient recovery. Research has shown that higher concentrations of protons in the cells actually provide necessary stimuli for improving the muscle-buffering capacity (5)(14). HIIT, therefore, is a sufficient training strategy for favorable buffering-capacity adaptations.
Increased Monocarboxylate Transporters
Cells have a means for remedying cellular acidosis, in other words, lactic acid and proton buildup. Monocarboxylate (MCT) transporters are proteins found on the cell membranes that facilitate the efflux of lactate and proton molecules from the intracellular environment into the blood. MCT is a co-transporter in that it collectively transports a molecule each of lactate and proton out of the cell. Exercise and training lead to increased MCT, and therefore an associated benefit in greater capacity for removal of excess lactate and protons from the cell (8). This provides a means for quicker recovery from exercise conditions that increase muscle lactate and proton concentrations (6)(7)(14).
Proper Recovery Effects
- Boosting tissue regeneration
- Decreasing fatigue
- Increasing supercompensation
- Enabling the use of heavy loads in training
- Decreasing the frequency and number of injuries
- Age – younger people recover faster
- Gender – female athletes recover slower
- Environment – hot/cold speeds up recovery
Meaning that exercising during notably cold or hot weather supplements recovery due to the resulting decrease in muscle inflammation during exposure to hot/cold temperatures - Type of muscle fibers – fast twitch fibers recover faster
- Type of exercise – endurance training requires longer recovery
- Nutrition – supplements recovery
References
- Kojda G., Hambrecht R. (2005) Molecular mechanisms of vascular adaptations to exercise. Physical activity as an effective antioxidant therapy?
- Gomez- Pinilla F. (2007) The influence of diet and exercise on mental health through hormetic.
- Bi L, Triadafilopoulos G. (2003) Exercise and gastrointestinal function and disease: and evidence-based review of risks and benefits.
- Wahid A, Manek N, Nichols M, Kelly P, Foster C, Webster P, Kaur A, Friedemann Smith C, Wilkins E, Rayner M, et al. (2016) Quantifying the Association Between Physical Activity and Cardiovascular Disease and Diabetes: A Systematic Review and Meta-Analysis.
- Lippincott Williams & Wilkins (2013), American College of Sports Medicine. ACSM’s guidelines for exercise testing and prescription
- Harsh Patel, Hassan Alkhawam, Timothy J Vittorio (2017) Aerobic vsanaerobic exercise training effects on the cardiovascular system
- Wasserman K. (1986) The anaerobic threshold: definition, physiological significance and identification.
- Powers, S.K. & Howley, E.T. (2009). Exercise Physiology: Theory and Application to Fitness and Performance (7th ed.). New York: McGraw-Hill Companies.
- Ivy, J.L. (2004). Regulation of muscle glycogen repletion, muscle proteins synthesis and repair following exercise.
- Baker, J.S., McCormick, M.C. & Robergs, R.A. (2010). Interaction among skeletal muscle metabolic energy systems during intense exercise.
- Thomas, C. et al. (2012). Effects of acute and chronic exercise on sarcolemmal MCT1 and MCT4 contents in human skeletal muscles.
- Bishop, D., Girard, O. & Mendez-Villanueva, A. (2011). Repeated-sprint ability – part II: Recommendations for training.
- Sportscicollective.com: Bompa, T. O., & Haff, G. G. (2009). Periodization: Theory and methodology of training. Human Kinetics Publishers., Issurin, V. B. (2010). New horizons for the methodology and physiology of training periodization. Sports medicine, 40(3), 189-206., – Brezhnev, Y. V., Zaitsev, A. A., & Sazonov, S. V. (2011). To the analytical theory of the supercompensation phenomenon.
- Bishop, D. et al. (2008). Effects of high-intensity training on muscle lactate transporters and post-exercise recovery of muscle lactate hydrogen ions in women.
- Rhea MR Ball SD Phillips WT Burkett LN. (2002) A comparison of linear and daily undulating periodized programs with equated volume and intensity for strength.
- Selye H. (1974) Stress without distress.
- Rhea MR Alderman BL. (2004) A meta‐analysis of periodized versus non- periodized strength and power training programs.
- Plisk SS Stone MH. (2003) Periodization strategies.
- Rhea MR Alvar BA Burkett LN Ball SD. (2003) A meta‐analysis to determine the dose response for strength development.
- Daniel Lorenz, DPT, PT, LAT, CSCS and Scot Morrison, PT, DPT, CSCS (2005), Current concepts in periodization of strength and Conditioning for the sports physical therapists
- Bompa (1999) Periodization Training for Sports, Human Kinetics.